The world health organization (WHO) keeps statistics on what kills the most people throughout the world every year. Heart disease or stroke is generally the most prevalent cause of death no matter what country you live in. On the other hand, most people in Western Europe or North America don’t die from malaria. But if you look at other parts of the world this statistic changes radically. Malaria is one of the greatest plagues to afflict humanity. The vast majority of malaria victims live in poor countries in Africa, Southeast Asia and South America. The numbers are simply staggering. According to the World Health Organization, 3.2 billion people remain at risk for malaria—that’s about half the population of the planet. About 88% of the estimated cases and 90% of the deaths occur in sub-Saharan Africa, followed by Southeast Asia (10%), the Eastern Mediterranean region (2%) and Central and South America (<1%). Deaths occur mostly in children under the age of five, pregnant women or immunodeficient people. Malaria continues to kill over three-times as many people as all the armed conflicts in the world put together. In 2015, for example, there were an estimated 631,000 deaths resulting from malaria, compared with an estimated 167,000 deaths due to armed conflicts. Some 200 million people were actually infected with malaria. And, although there are effective treatments for the disease, things are far from being under control. After a decade of dramatic reductions in malaria mortality, the 2017 World Malaria Report estimated that mortality rates stalled in Southeast Asia, the Western Pacific and Africa and increased in the Eastern Mediterranean and the Americas between 2015 and 2016. So, as we struggle to find better ways for controlling malaria, the plague is still very much with us—and it always has been.
The spread of malaria to any individual is due to the bite of a mosquito, the “vector” that injects the pathogenic agent responsible for the disease into the human blood stream. The organism that causes malaria is the protozoan Plasmodium spp. There are actually several closely related protozoan parasites that can cause the disease. Moreover, there are also different species of mosquitoes that can spread malaria, though only females of the Anopheles genus can transmit human malaria.
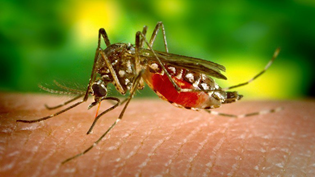
Anopheles mosquitos actually feed on nectar—blood isn’t what they eat. However, it turns out that there are nutrients in human blood—protein and iron—that are vital for a female Anopheline mosquito to be able to develop her eggs. This is the reason why she will drink about 3 millionths of a liter of blood when she bites a human victim. If the victim is infected with Plasmodium, the parasite will enter the mosquito and then be transmitted to another host the next time the mosquito bites somebody else. Approximately 100 species of Anopheline mosquitoes can transmit the five species of Plasmodium capable of causing human malaria. The organisms involved are 5 species of the protozoan Plasmodium—P.vivax , P. falciparum and P. malariae being the most common forms of the disease in humans. Related protozoa can infect other animals including birds, reptiles and even fish. Mosquitoes are one of humanity’s most deadly enemies. Some of the most important human diseases are borne by mosquitoes including malaria (Anopheles), filariasis (Culex, Mansonia and some Anopheles spp.), viral encephalitides (Culex) as well as the viral diseases yellow fever, dengue fever, Zika and chikungunya, which are transmitted by Aedes aegypti mosquitoes.
There are several treatments for human malaria. The drug quinine, obtained from the bark of the cinchona tree, was first “discovered” by the Spanish following their conquest of Peru in the 17th century. Quinine is certainly effective at targeting Plasmodium parasites and killing them, although even today nobody is completely sure as to its mechanism of action. Following the establishment of the pharmaceutical industry in the 19th century, synthetic drugs like chloroquine were produced. These synthetic drugs had quinine-like actions and became the drugs of choice for effectively treating malaria for many years. Then, in the 20th century, the Chinese scientist Tu Youyou isolated the anti-malarial drug artemisinin from Artemisia annua, the sweet wormwood plant. Tu became the first Chinese woman to win the Nobel Prize. Artemisinin proved to have potent effects against malaria and work by a completely different mechanism of action than chloroquine. Combinations of the two types of drugs are currently the state of the art for treating malaria and have certainly saved the lives of millions of people. However, the rise of drug resistance has suggested that these drugs may not be as effective in the future and novel approaches for treating malaria are urgently required.
A different kind of approach targets mosquitoes themselves rather than treating the protozoan pathogen in infected individuals. Ridding the environment of mosquitoes would also have the advantage of getting rid of several mosquito-associated diseases in addition to malaria. Spraying insecticides on mosquito-infested areas is one approach to this problem. Insecticides such as pyrethroids, organophosphates and carbamates are used for this purpose but, in recent years, mosquitoes in sub-Saharan Africa have gained sufficient resistance to render chemical insecticides largely ineffective. The various insecticides work by poisoning the nervous system of the mosquito. Organophosphates inhibit the activity of the enzyme cholinesterase and so block the metabolism of the neurotransmitter acetylcholine. Pyrethroids, originally isolated from the chrysanthemum plant, alter the properties of the voltage-dependent sodium channels that allow nerves to conduct action potentials. The ultimate effects of these insecticides is to produce paralysis of mosquitoes. However, in addition to the problem of developing drug resistance, most traditional insecticides are not specific to mosquitoes and can destroy other “useful” insects and sicken animals including humans. Nevertheless, based on the considerable success using insecticides, it is clear that poisoning the mosquito nervous system might be an interesting approach for the future development of new types of mosquitocidal or malariacidal biomolecules.
One important fact to note is that mosquitoes have their own problems with parasites. For example, Metarhizium is a genus of entomopathogenic fungi, that is fungi that can colonize different insects and kill them. This has led to the idea that such fungi might actually be used for insect-control purposes. For example, a specific grasshopper pathogen, Metarhizium acridum, was identified as a most promising candidate agent for dealing with insect infestations. The fungus was turned into a commercial product called “Green Muscle” which was first employed in 2000 in Madagascar, Niger, Senegal and Sudan. In May 2009, it successfully contained an outbreak of red locusts that were threatening crops in East and Southern Africa.
In light of such positive results, one current idea is that an appropriate strain of Metarhizium might also be used to kill mosquitoes. M. pingshaense, for example, has a narrow host range suggesting that its effects might be restricted to mosquitoes without poisoning other important insects such as honeybees. Results using this approach for killing mosquitos have also been promising. Initial studies showed that spores from a specific Metarhizium strain could effectively reduce mosquito populations. However, killing the mosquitoes required very large quantities of fungal spores and usually took a couple of weeks to work. Really, if we want to stop mosquitoes spreading malaria, it would be best to have something that worked quickly, before the mosquito had time to pass on its deadly cargo. In other words, something like M. pingshaense is potentially a great way of introducing a pathogenic agent into mosquitoes but we need a way to pep it up a bit and make it work faster. Perhaps, as is commonly done these days, one might genetically modify the fungus so that it produces something that, like an insecticide, is toxic to mosquitoes?
This seems like a very reasonable idea. So which toxic agent should one use for genetically engineering a mosquitocidal fungus? Perhaps something that is already employed for this purpose in Nature? In fact, the number of naturally occurring toxins that are potentially available for this purpose is absolutely colossal. One source that could possibly yield a suitable toxin would be an animal venom. There are really two reasons why animals produce venoms. One reason is to produce “nociception”, what humans would refer to as pain, as a defensive measure against predators. The second reason is to disable or kill prey. The range of organisms that produce toxic venoms for capturing prey or for defense against potential predators is surprisingly diverse and includes eubacteria, arthropods, molluscs, cnidarians, plants, and numerous vertebrates. Really, producing a toxic venom is the “rule” rather than the “exception” for most animals. Even some mammals such as the duck-billed platypus are venomous. However, the precise molecular composition of venoms is usually extremely complex and, as far as their detailed characterization is concerned, we have only really just scratched the surface. The venom compositions of snakes, cone snails and scorpions have been investigated in some detail and have been shown to contain many different kinds of molecules. These include enzymes that break down the walls of cells causing them to lyse and create inflammatory reactions. However, peptides, in particular, are often the venom components that produce effects such as pain and paralysis by engaging specific receptors in the nervous system of intoxicated victims. And it’s not just one toxic peptide that is found in each venom. Usually, there is a vast array of these molecules which act simultaneously and synergistically on different targets. Evolution really wanted to make sure that venoms did the job they were designed for.
So, would the expression of insect toxins by Metarhizium spp. increase its ability to kill mosquitoes? The chemical insecticides traditionally used in mosquito-control programs are directed against neuronal voltage-gated sodium channels, as are many insect-specific neurotoxins derived from arthropods. These include the scorpion toxin AaIT, a single chain neurotoxic polypeptide derived from the venom of the Buthid scorpion Androctonus australis hector that targets nerve sodium channels, and has been approved for use as a potential insecticide in several countries. A genetically modified strain of the entomopathogenic fungus Metarhizium anisopliae expressing AaIT was found to kill mosquitoes faster and at lower spore doses than normal “wild-type” fungus, illustrating the potential utility of this approach. But perhaps other toxins could be used to produce an even more deadly fungus?
With respect to further improving Metarhizium spp. for the control of insects, considerable current interest has centered on the use of toxins from extremely venomous Australian funnel-web spiders. Spiders are probably the most diverse group of venomous creatures on the planet. Spiders evolved from a common ancestor in the Devonian period around 400 million years ago and currently comprise over 100,000 different species in more than 3,500 genera. One study used a conservative estimate of approximately 50 peptide toxins per spider venom obtained from mass spectrometric analysis of 55 tarantula venoms and a conservative species count of 80,000 different kinds of spiders, leading to an estimated total of 4 million spider venom toxin polypeptides. However, this estimate may be low. A recent study of 18 venoms from lethal Australian funnel-web spiders using the most up-to-date mass spectroscopy technology revealed the presence of approximately 800 peptides in female venoms and 400 peptides in male venoms. The major components of spider venoms are typically small peptide toxins of mass 2–9 kDa that can be grouped into three categories:
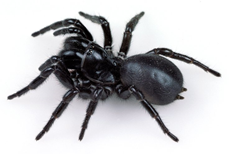
(i) cytolytic peptides, typically of mass 2–4 kDa (ii) medium-sized neurotoxic peptides, typically comprising 35–45 amino acid residues (iii) larger polypeptides (5–9 kDa), mostly of unknown function. Some of these spider toxins are extremely potent, producing paralysis of prey at very low concentrations, making them attractive possibilities as agents that might be genetically engineered into entomopathogenic fungi. A good candidate would have potent activity on mosquitoes but have no effects on humans.
Funnel-web spider venoms contain toxins such as the ω-hexatoxins (formerly known as ω-atracotoxins) such as ω-HXTX-Hv1a, peptides comprising some 37 residues that were first isolated from the venom of the lethal Blue Mountain funnel-web spider Hadronyche versuta. These toxins contribute significantly to prey immobilization by virtue of their ability to specifically block insect, but not vertebrate, voltage-gated calcium channels, a target that has not been previously utilized by commercial insecticides. Furthermore, in contrast to ω-HXTX-Hv1a, the venoms also contain κ-HXTX-Hv1c which is a potent and specific blocker of calcium-activated potassium channels, another unique target expressed by nerves. Finally, a family of “hybrid” toxins, the ω /κ-HXTX family, was found in two of the species examined (A. robustus and H. versuta ). ω/κ-HXTX-Hv1 was found to be highly insecticidal resulting from a synergistic effect on insect voltage-gated calcium channels and calcium-activated potassium channels. Indeed, this toxin has been approved by the Environmental Protection Agency (EPA) under the trade name VersitudeTM for its potential insecticidal actions. Because it has already been approved by the EPA, it is an attractive possibility for arming Metarhizium spp. This makes very good sense from a biological point of view. The muscles that allow the mosquito to fly and move are all under the control of nerves. These nerves function by firing electrical signals called action potentials and releasing chemical neurotransmitters that allow the muscles to contract or relax. Action potentials can only be formed when ion channels that conduct sodium and potassium ions are activated in precisely the correct sequence. Furthermore, neurotransmitters can only be released from nerves when calcium channels open and close correctly. Hence, peptide toxins that disrupt the normal functions of these channels in the mosquito nervous system would be predicted to have a paralyzing effect on these insects.
To find out whether the efficacy of mosquito killing could be enhanced further, investigators prepared genetically engineered Metarhizium pingshaense that expressed one of several insect-specific neurotoxins with different mechanisms of action. The toxins tested were μ-CUTX-As1a, a toxin from the venom of the Trap-door spider which specifically blocks insect voltage-dependent sodium channels; the funnel-web spider toxin ω/κ-hexatoxin-Hv1a (“Hybrid”, VersitudeTM) which blocks neuronal calcium and calcium-activated potassium channels; the funnel-web spider toxin ω-hexatoxin-Hv1a (Hv1a) that blocks calcium channels; and the Hobo spider toxin U1-AGTX-Ta1a. In a series of experiments, toxins were genetically engineered so that they would be expressed by Metarhizium pingshaense under control of a mosquito hemolymph-specific promoter. Hemolymph is the fluid that mosquitoes have instead of blood. So engineering the fungus in this way means that the toxic peptides would only be made when the fungus was in contact with the mosquito “blood stream”, ensuring that it was targeted appropriately. The investigators observed that several of the toxins were effective in enhancing the killing effects of the fungus. Fewer fungal spores were required and mosquitoes died much more quickly. The funnel-web hybrid toxin, also known as VersitudeTM, was chosen for further study as the US EPA had already approved it for use as a standalone insect-control agent. Encouragingly, further tests showed that the transgenic fungus only killed mosquitoes and had no effect on honeybees.
But how would the new strain of fungus perform when tested outside of the laboratory? A recent paper published in the journal Science reported the results of such a trial. To start with, the investigators had to choose a site to carry out their experiments. The African country of Burkina Faso has a serious malaria/mosquito problem. Indeed, the country has one of the highest rates of malaria in the world and many of the mosquitoes there have become resistant to traditionally used insecticides. The authorities in Burkina Faso are extremely keen to facilitate research that will help to eradicate their mosquito problem. To this end, they have established a research facility called the MosquitoSphere where experimental treatments such as genetically modified organisms can be tested. The MosquitoSphere facility consists of separate huts which can be infested with mosquitoes and any potential treatment can be tested to see if it is effective.
It turns out that after biting their victims, female mosquitoes like to take a little nap and, in particular, like to do this on black colored surfaces. So the investigators put 500 female and 1000 male mosquitoes in each test hut. They also hung the walls of each hut with black sheets covered in sesame oil impregnated with various test organisms. One was ordinary M. pingshaense. Another hut had M. pingshaense that expressed the VersitudeTM spider toxin. A third hut had no fungus in it at all (“control hut”). The investigators then left things to cook for 45 days. The results were very impressive. After this time, there were as many as 2,500 adult mosquitoes in the control hut, roughly 700 in the hut with the normal fungus, but only 13 in the hut with the genetically modified fungus. The researchers say that the fungus should be relatively easy to contain in Nature because its spores are sticky and so will not be easily dispersed by the wind. Importantly, further tests demonstrated that the fungus didn’t harm other beneficial insects, including honeybees.
These experiments are ingenious and the results are very encouraging. One can certainly envisage a time when the genetically engineered fungus will be impregnated into mosquito nets used by people in malaria-infested areas of the world and, if the results so far are anything to go by, this may be an excellent way of controlling the mosquito population. However, there is still a great deal of public suspicion directed against the use of genetically engineered organisms that are set to be released into the wild. Clearly, a number of assurances will have to be forthcoming before that actually happens. It should also be noted that this is not the only novel approach to mosquito management that is under consideration these days. Another interesting approach is that of the “gene drive” in which genetically altered mosquitoes express a mutation in the doublesex gene that is important for egg development. The way that the gene is altered means that its dissemination throughout the mosquito population is “super-Mendelian” and so it spreads very rapidly to the point that the entire population becomes sterile and stops reproducing. Here again, the technique has been shown to work successfully in the laboratory but faces many hurdles before it is tested in the wild. Nevertheless, both the gene drive and genetically engineered fungus techniques are extremely innovative and are examples of new ways for potentially combating mosquito-associated diseases. However, even if these methods are ultimately successful, history has shown us that microbes and insects can be relied on to find ways of eventually developing resistance to anything we throw at them, likely ensuring that the deadly game of “cat and mouse” will continue for the foreseeable future.
FURTHER READING:
Transgenic Metarhizium rapidly kills mosquitoes in a malaria-endemic region of Burkina Faso.
Lovett B, Bilgo E, Millogo SA, Ouattarra AK, Sare I, Gnambani EJ, Dabire RK, Diabate A, St Leger RJ. Science. 2019 May 31;364(6443):894-897. doi: 10.1126/science.aaw8737. PMID: 31147521